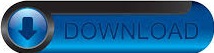
HK, Hexokinase G6PDH, glucose-6-phospate dehydrogenase PGLS, 6-phosphogluconolactonase 6PGDH, 6-phosphogluconate deydrogenase. The portion of the pathway illustrating entry of δ-gluconolactone and metabolism to 6PG are highlighted in blue and enclosed in a box. However, to date, studies examining the contribution of PGLS to redox maintenance or tumor proliferation in cancer are lacking. Glucose-6-phosphate dehydrogenase and 6-phosphogluconate dehydrogenase are, therefore, therapeutic targets in cancer ( 16, 19, 20). In addition, these enzymes have been identified as the major sources of NADPH, which, in turns, plays an essential role in maintaining glutathione (GSH) in the reduced state and thereby, combating oxidative stress ( 11, 12, 16, 18). Several studies have identified essential roles for glucose-6-phosphate dehydrogenase and 6-phosphogluconate dehydrogenase in the generation of precursors for ribonucleotide synthesis, and thereby, nucleic acid synthesis ( 12, 16, 17). Subsequent formation of ribulose-5-phosphate occurs via oxidative decarboxylation of 6PG by 6-phosphogluconate dehydrogenase with the concomitant generation of NADPH. 6-phospho-δ-gluconolactone, which is in equilibrium with 6-phospho-γ-gluconolactone ( 14, 15), is then hydrolyzed by 6-phosphogluconolactonase (PGLS) to generate 6-phosphogluconate (6PG). The PPP branches from glycolysis at the level of glucose-6-phosphate, which is oxidized by glucose-6-phosphate dehydrogenase to 6-phospho-δ-gluconolactone while reducing NADP + to NADPH (see Figure 1). Although less is known about glucose metabolism through the pentose phosphate pathway (PPP), emerging evidence points to upregulation of PPP flux in tumor tissues, including in glioblastomas ( 11– 13). The best studied metabolic hallmark in cancer is the Warburg effect wherein tumor cells increase glucose uptake and concomitant lactate production even under aerobic conditions ( 10). All tumor cells, including glioblastomas, acquire oncogenic mutations that facilitate rewiring of metabolic pathways to allow for the increased demand for biosynthetic intermediates and redox metabolites needed to support uncontrolled proliferation ( 8, 9). Nutrient uptake and metabolism are tightly regulated in normal somatic cells. Metabolic reprogramming has emerged as a fundamental hallmark of cancer ( 7– 9). In order to improve patient outcomes, there is a need to identify biological processes that are critical for tumor proliferation as well as non-invasive imaging methods that can inform on tumor biology and response to therapy ( 6). Nevertheless, overall patient survival remains short at ~14.6 months ( 4, 5). The current standard of care for glioblastomas consists of maximal safe surgical resection combined with radiotherapy and chemotherapy with temozolomide (TMZ) ( 4, 5). They account for approximately half of all glioma cases and have a very dismal prognosis with a 5-year patient survival rate of <7% ( 1). High-grade (grade IV) tumors that are isocitrate dehydrogenase wild-type are now considered primary glioblastomas. In 2016, the World Health Organization revised the criteria for glioma classification to include molecular characteristics ( 2, 3). Gliomas are traditionally classified by histology, in increasing order of malignancy from grade I to grade IV. Gliomas are the most common form of adult-onset primary malignant brain tumors with 25,130 cases expected to be diagnosed in 2021 ( 1). Longitudinal 2D EPSI studies of hyperpolarized δ-gluconolactone metabolism and T2-weighted magnetic resonance imaging (MRI) were performed in rats bearing orthotopic U251 tumors following treatment with TMZ to examine the ability of hyperpolarized δ-gluconolactone to report on treatment response. 13C 2D echo-planar spectroscopic imaging (EPSI) studies of hyperpolarized δ-gluconolactone metabolism were performed on rats bearing orthotopic glioblastoma tumors or tumor-free controls on a 3T spectrometer. Hyperpolarized δ-gluconolactone metabolism to 6PG was assessed in live cells treated with the chemotherapeutic agent temozolomide (TMZ) or with vehicle control. Clonogenicity assays were used to assess the effect of PGLS silencing on glioblastoma proliferation. To interrogate the function of PGLS in redox, PGLS expression was silenced in U87, U251 and GS2 glioblastoma cells by RNA interference and levels of NADPH and reduced glutathione (GSH) measured.
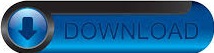